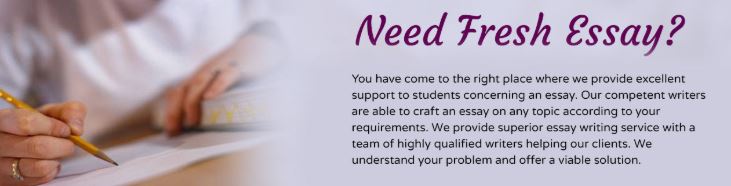
Clinical Neurophysiology 126 (2015) 170–179
Contents lists available at ScienceDirect
Clinical Neurophysiology
journal homepage: www.elsevier .com/locate /c l inph
Abnormal cortical activation in females with acute migraine: A magnetoencephalography study
http://dx.doi.org/10.1016/j.clinph.2014.03.033 1388-2457/� 2014 International Federation of Clinical Neurophysiology. Published by Elsevier Ireland Ltd. All rights reserved.
⇑ Corresponding author. Address: Department of Neurology, Nanjing Brain Hospital, Nanjing Medical University, Guang Zhou Road 264, Nanjing, Jiangsu 210029, PR China. Tel.: +86 025 82296208; fax: +86 025 83719457.
E-mail address: [email protected] (X.S. Wang).
Huai T. Ge a, Hong X. Liu a, Jing Xiang b, Ai L. Miao a, Lu Tang a, Qing S. Guan a, Ting Wu a, Qi Q. Chen c, Lu Yang c, Xiao S. Wang a,⇑ a The Department of Neurology, Nanjing Brain Hospital, Nanjing Medical University, Nanjing, Jiangsu, PR China b The MEG Center, Division of Neurology, Cincinnati Children’s Hospital Medical Center, 3333 Burnet Avenue, Cincinnati, OH 45220, USA c The MEG Center, Nanjing Brain Hospital, Nanjing, Jiangsu, PR China
a r t i c l e i n f o h i g h l i g h t s
Article history: Accepted 31 March 2014 Available online 16 April 2014
Keywords: Migraine Magnetoencephalography (MEG) Magnetic source imaging (MSI)
� It is well known that cerebral cortex plays a key role in migraine attacks and movement makes migraine headaches worse; however, its neural mechanism is poorly understood.
� Finger movements produced cortical hyperexcitability in females with migraine during migraine attack in a frequency range of 5–1000 Hz.
� Neuromagnetic signal may help to identify neurophysiological biomarkers for studying mechanisms of migraine using MEG and may facilitate to develop new therapeutic strategies for migraine.
a b s t r a c t
Objective: The objective of this study was to investigate functional abnormalities of the brain in females with migraine using magnetoencephalography (MEG) and a finger-tapping task. Methods: Twenty-nine female patients with migraine (aged 16–40 years) and age- and gender-matched healthy controls were studied with an MEG system at a sampling rate of 6000 Hz. MEG recordings were performed during an attack in migraineurs with and without aura. Neuromagnetic brain activation was elicited by a finger-tapping task. The latency and amplitude of neuromagnetic responses were analyzed with averaged waveforms in the frequency range of 5–100 Hz. The Morlet wavelet and beamformers were used to analyze the spectral and spatial signatures of MEG data from subjects in two frequency ranges of 5–100 and 100–1000 Hz. Results: The latency of motor-evoked magnetic fields evoked by finger movement was significantly prolonged in migraineurs as compared with controls. Neuromagnetic spectral power in the motor cortex in migraineurs was significantly elevated. There were significantly higher odds of activation in 5–30, 100–300 and 500–700 Hz frequency ranges in the ipsilateral primary motor cortices and the supplementary motor area in migraineurs as compared with controls. Conclusions: Neuromagnetic signal abnormalities in this study suggest cortical hyperexcitability in females with migraine during migraine attack, which could be measured and analyzed with MEG signal in a frequency range of 5–1000 Hz. Significance: These findings may help to identify neurophysiological biomarkers for studying mechanisms of migraine, and may facilitate to develop new therapeutic strategies for migraine by alterations in cortical excitability. � 2014 International Federation of Clinical Neurophysiology. Published by Elsevier Ireland Ltd. All rights
reserved.
1. Introduction
Migraine is a common disabling headache disorder character- ized by ictal episodes of moderate-to-severe focal pulsating
H.T. Ge et al. / Clinical Neurophysiology 126 (2015) 170–179 171
headache (Coppola and Schoenen, 2012). Migraine sufferers are typically hypersensitive to visual (photophobia), auditory (phono- phobia), and sensory (cutaneous allodynia) stimuli during migraine attack (Gelfand et al., 2010). Migraine frequently begins in childhood and the prevalence increases in adolescence and early adult life. The prevalence of migraine is the same in boys and girls before adolescence; it increases predominantly in females com- pared with males after puberty (Hershey, 2010). According to the World Health Organization, migraine has become one of the 20 most disabling diseases (Leonardi et al., 2005). The occurrence of frequent headaches can have a significant impact on the human quality of life (Gladstein and Rothner, 2010). It is therefore a public health problem having a great impact on both the individuals and the society.
The neurobiological mechanism underlying migraine attacks remains unclear. There are evidences that the cerebral cortex plays a key role in migraine attacks (Hershey, 2005, 2010; Hershey et al., 2007; Pietrini et al., 1987). Bowyer and colleagues have found that patients with migraine have cortical hyperexcitability (Bowyer et al., 2005). Neurophysiological reports have shown that migraine is associated with abnormal excitability in visual and somatosen- sory cortices (Bowyer et al., 2001; Gunaydin et al., 2006; Lang et al., 2004). Recent magnetoencephalography (MEG) studies revealed that children with migraine, during their headache attacks, have significantly prolonged latencies of neuromagnetic activation in a low-frequency range, with increased spectral power in high-frequency ranges (Xiang et al., 2013; Wang et al., 2010). The spread of abnormal brain activation triggered by movements seems to play an important role in the cascade of pediatric migraine attacks (Xiang et al., 2013). As age is an important factor in headache severity, duration, frequency, and subsequent second- ary disability (Bohotin et al., 2002), it remains unknown if adult patients with migraine are also associated with motor cortical dys- function during migraine attacks.
The involvement of motor cortex in migraine has drawn atten- tion recently (Esposito et al., 2012; Conforto et al., 2012; Wang et al., 2010). It is well known that movement makes migraine headaches worse, and one subset group of migraine patients shows hemiplegia (Scherer et al., 1997); however, its neural mechanism is poorly understood. Reports on nonfamilial migraine with unilate- ral motor symptoms (MUMSs) showed that a syndrome of severe migraine with accompanying give-way weakness is common in tertiary care headache centers (Young et al., 2007). An increasing list of transcranial magnetic stimulation (TMS) reports indicates that motor cortical dysfunction may play an important role in the pathogenesis of attacks of migraine (Aurora et al., 1999; Conforto et al., 2012; Conte et al., 2010; Fumal et al., 2003; Khedr et al., 2006; Maertens et al., 1992). From a therapeutic point of view, high-frequency repetitive TMS (rTMS) of the motor cortex can normalize aberrant intracortical inhibition during migraine (Brighina et al., 2010). Neurophysiologically, rTMS of the motor cortex can also modulate pain-related evoked responses in migraine (de Tommaso et al., 2010). Of note, a clearer understand- ing of motor cortical activation in patients with migraine is scien- tifically and clinically important.
The objective of the present study was to investigate motor cor- tical dysfunction in female adults with migraine using MEG and a finger-tapping task. We focused on females because the majority of patients with migraine are females and the ratio of migraine in female and male is approximately 3:1 (Hershey, 2012; Buse et al., 2012). A finger-tapping task was used because the task could activate the motor cortex without causing significant head move- ment. Our central hypothesis is that neuromagnetic activation in the motor cortex in females with migraine is significantly altered as compared with controls. To our knowledge, this is the first MEG study focusing on abnormalities of brain activation in the
5–1000-Hz frequency range in female adults with migraine using waveforms, spectrograms, and magnetic source imaging (MSI). Findings of this study may contribute to a better understanding of the mechanisms of migraine, and to the development of new therapeutic strategies in the future.
2. Methods
2.1. Subjects
Twenty-nine female patients with acute migraine (mean age: 30.59 years; standard deviation: 5.75 years, range: 16–40 years) were recruited from Nanjing Brain Hospital (see Table 1). Six of them had aura, while the remaining did not. Twenty-nine age- matched healthy female controls (mean age 30.59 years; standard deviation 5.75 years, range: 16–40 years) were recruited. All sub- jects >18 years of age signed a consent form. For subjects <18 years of age, their parents/guardians signed a consent form. This study and the consent form were approved by the Institutional Review Board at Nanjing Brain Hospital before undertaking the study.
The inclusion criteria were (1) migraine with or without aura as defined in the International Classification of Headache Disorders, 2nd Edition (ICHD-II) and (2) no other neurologic disorder. Con- trols were recruited to match the migraine subjects for age and gender and met inclusion criteria of (1) no history of neurologic disorder, headache, or brain injury and (2) age-appropriate hearing and hand movement. Exclusion criteria for all participants were (1) presence of an implant (e.g., braces or pacemaker) which could produce visible magnetic noise in the MEG data; (2) demonstration or expression of noticeable anxiety and/or inability to communi- cate readily with personnel operating the MEG equipment; (3) inability to keep still; and (4) subjects with claustrophobic tenden- cies or pregnancy (for MRI scans).
2.2. Tasks
All subjects were asked to perform a brisk index finger tapping with either right or left index finger while hearing a cue (500 Hz, 30 ms square tone). Subjects were instructed to press the response button with the index finger that was ipsilateral to the tone pre- sented (Wang et al., 2010; Xiang et al., 2001, 2013). Other body parts were kept still and the eyes kept open, fixed to an arbitrary target during the paradigm. A trigger was sent to the MEG system from the response box when the button was pressed. The stimuli consisted of 200 trials of square tones and 100 trials per ear, and were presented randomly through a plastic tube and earphones. The stimulation presentation and response recording were accom- plished with BrainX software, which was based on DirectX (Micro- soft Corporation, Redmond, WA, USA) (Huo et al., 2011; Wang et al., 2010; Xiang et al., 2001, 2013). The entire procedure took about 15 min.
2.3. MEG recording
The MEG signals were recorded in a magnetically shielded room (MSR) using a whole head CTF 275-Channel MEG system (VSM MedTech Systems, Inc., Coquitlam, BC, Canada) in the MEG Center at Nanjing Brain Hospital. Before data acquisition commenced, all subjects were asked to remove all possible metals from their body. After that, electromagnetic coils were attached to the nasion and left and right preauricular points of the subject. These three coils were subsequently activated at different frequencies for measuring subjects’ head positions relative to the MEG sensors. Subjects were asked to lie still comfortably in a supine position during the entire procedure, with arms resting on either side. The sampling rate of
Table 1 Clinical features of the migraine patients.
Parameters Statistics migraine controls
Age (years) (mean ± SD) 30.59 ± 5.75 30.59 ± 5.75 Gender (female/male) 29/0 29/0 Handedness (right/left) 26/3 25/4 Classification of migraine (with aura/without aura) 6/23 N Attack frequency of migraine per months (mean ± SD) 2.41 ± 1.57 N Duration of the attack (h) (mean ± SD) 16.82 ± 13.28 N Severity of headache (on scale: 0–10) (mean ± SD) 5.72 ± 1.28 N Years of suffering from migraine (mean ± SD) 10.81 ± 5.35 N Average of the age of onset (mean ± SD) 16.55 ± 5.69 N Pain feature
Throbbing 18 Pressure 14 Stabbing 4 Sharp 1 Other 2 N
Location Bilateral 11 Unilateral 19 N
Medication for treatments before MEG tests (number of subjects; multiple drugs/nutraceuticals might be used) No-drug 15 Ibuprofen 18 Dihydroergotamine(DHE) 4 Sibelium 14 Percocet 9 Levetiracetam 1 Topiramate 2 Valproate sodium 5 Coenzyme Q10 11
Abbreviations: SD, standard deviation; N, normal. No-drug: subjects claimed that they did not use any medications for at least 1 week prior to MEG tests.
172 H.T. Ge et al. / Clinical Neurophysiology 126 (2015) 170–179
the MEG recordings was 6000 Hz per channel. An acquisition win- dow was set to 3000 ms per trial, with 2000 ms per trigger. The trigger was sent to the MEG system from a response when a button was pressed. Data were recorded with a noise cancelation of third- order gradients and without online filtering. Subjects were asked to remain still during all procedures. The head positions were mea- sured at the beginning and the end of procedures. If head move- ment during a recording was beyond 5 mm, the set was indicated as ‘‘bad’’ and an additional trial was recorded.
2.4. Magnetic resonance imaging scan
The anatomical image data were obtained for all subjects using a 1.5 MRI (Singa, GE, USA). Three fiducial markers were placed in locations identical to the positions of the three coils used in the MEG recordings. An accurate co-registration of the MEG and mag- netic resonance imaging (MRI) data was thus acquired with the three landmarks. Subsequently, all anatomical landmarks digitized in the MEG study were made identifiable in the MRI.
2.5. Data analysis
2.5.1. Morphology The MEG data were manually averaged after the removal of eye
movement and other artifacts. The averaged MEG data were pre- processed by removing the direct current (DC) offset based on the pre-trigger baseline, then the movement-evoked magnetic fields (MEFs) were obtained. A low-pass filter (100 Hz) and a high-pass filter (5 Hz) were applied to the manually averaged MEG data. The latencies and the peak amplitudes of the averaged evoked magnetic fields (deflections) were automatically measured by putting the cursor on the peak of each recognizable component.
2.5.2. Time–frequency analysis The time-domain MEG data were transformed to spectrograms,
and the time–frequency representations of MEG data using the
Morlet continuous wavelet transform. The Morlet continuous wavelet algorithm is described as the following equation:
Wðt; sÞ ¼ crp� 1 4e�
1 2t
2 ðeirt � jrÞ
Because frequency–temporal resolution changes with the sigma value and the wavelet transform is sensitive to time but not fre- quency at high-frequency signals, the sigma value was dynamic, changing according to the frequency range. The present study focused on the frequency bands of 5–100 and 100–1000 Hz. Neuro- magnetic signals <5 Hz were discarded because the computation of low-frequency components needed more data points that might include premotor activations such as readiness magnetic fields. Our study focused on the spectral power changes in 0–200 ms fol- lowing finger tapping, which include all components of MEFs obtained from the time-domain waveform in the previous section. Each trial of MEG data was transformed from time-domain wave- form to frequency domain spectrogram individually. After all trials were transformed to spectral data, all trials were then averaged as an accumulated spectrogram. Polarity spectrogram was obtained after the magnetic polarity was color coded into the accumulated spectrogram. Multichannel spectrogram MEG data were then ana- lyzed with spectral contour map for visualizing the spatial distri- bution of brain activations elicited by finger movements. We computed the spectral power using the root-mean-square (RMS) value, which was automatically and objectively measured by com- putation. To quantify frequency resolutions for frequency ranges of 5–100 and 100–1000 Hz, the frequency bins were 600. The fre- quency resolution was 6.315, 0.667 data points per hertz for the two frequency ranges.
2.5.3. Source location The sources of finger-tapping-elicited magnetic signals were
estimated with wavelet-based beamformer (Xiang et al., 2010, 2013). At each coordinate voxel in source imaging, our method computed coefficients Wh using the unaveraged MEG data with
Fig. 1. Magnetoencephalography (MEG) waveforms showing neuromagnetic acti- vation evoked by finger movement (Left and Right fingers) in a migraine subject (‘‘Migraine’’) and a healthy subject (‘‘Control’’) in a frequency band of 5–100 Hz. There are at least two responses in the movement-evoked magnetic fields (MEFs), ‘‘MEFI’’ and ‘‘MEFII’’. There are differences in the morphology of the MEG waveforms between migraine and control. The latencies of the first and second responses in migraine subjects are longer when compared with those in the controls. The ‘‘Trigger’’ indicates the start of finger tapping. The ‘‘MEFI’’ and ‘‘MEFII’’ indicate the first and second responses of MEFs, respectively.
H.T. Ge et al. / Clinical Neurophysiology 126 (2015) 170–179 173
the following equation:Wh ¼ C�1Bh=BTh C �1Bh, where C is the covari-
ance matrix of the MEG data and B is the forward solution for a unit current source at a location h. For beamforming analysis, multiple local spheres were fitted to each patient’s head model. A customer- designed program, MEG Processor (Cincinnati, OH, USA), was used to estimate magnetic sources for signals in frequency bands of 5– 30, 100–300, and 500–700 Hz and a time window of 0–200 ms. The frequency bands and time window were determined using our pilot data. The distributions of source power were displayed on the individual MRI images. To obtain the dynamic spatiotempo-
Table 2 The main dependent variables analyzed in the MEG spectrograms for ictal migraine subje
Analysis frequency Measure variables Movement finger
5–100 Hz Latency 1 (ms) Left Right
Latency 2 (ms) Left Right
Amplitude 1 (fT) Left Right
Amplitude 2 (fT) Left Right
Location Left
Right
Source power (fT/Hz) Left Right
100–1000 Hz Location Left
Right
Source power (fT/Hz) Left Right
Abbreviations: MA, primary motor area; SMA, supplementary motor area.
ral activity in the brain, sliding window was used in the source estimation.
2.5.4. Statistical analyses A two-sample paired t-test analysis was applied to the latencies
and amplitudes of post-movement peaks of MEF between migraine subjects and controls. v2 tests were used to analyze the locations of aberrant brain activation elicited by finger tapping between migraine and controls. The threshold of statistical significance for differences was set at p < .05. For multiple comparisons, the Bon- ferroni correction was applied.
3. Result
3.1. Clinical characteristics
The characteristics of the 29 patients with migraine are summa- rized in Table 1. Out of the 29 patients with migraine, 6 (20.7%) had aura; 26 (89%) had moderate-to-severe headache; and 19 (66%) subjects had unilateral headache attacks. Unfortunately, among the migraine subjects with aura, no one experienced the aura dur- ing the recordings. One subject had two recordings because her head movement was beyond 5 mm during the first recording.
3.2. Morphology
As shown in Fig. 1, there were at least two responses (deflec- tions) in the MEG waveforms recorded from all migraine patients and controls. These responses in the waveforms were termed as movement-evoked magnetic field (MEF)I and MEFII. The latencies of two responses evoked by left and right finger movements in migraine patients and controls are shown in Table 2. In comparison with controls, the latencies of MEFI and MEFII in migraine subjects were significantly delayed. In the analysis range for 5–100 Hz, there were no latency differences of the MEFI and MEFII between migraineurs with aura and without aura (MEFI: p > .05; MEFII: p > .05, see Table 3).
cts and controls.
Migraines Controls p Value
40.72 ± 7.19 27.61 ± 4.32 <.05 42.96 ± 9.09 27.53 ± 3.51 <.05 94.89 ± 10.25 78.79 ± 5.58 <.05
101.51 ± 9.53 80.71 ± 3.88 <.05 663.13 ± 147.06 706.71 ± 150.86 0.1 736.82 ± 161.69 703.76 ± 100.28 0.2 484.44 ± 126.20 525.08 ± 115.41 0.06 522.62 ± 97.88 493.47 ± 72.55 0.2 Right MA(29/29) Right MA(29/29) >.05 Left MA(10/29) Left MA(2/29) <.05 Right SMA(3/29) Right SMA(1/29) >.05 Left MA(29/29) Left MA(29/29) >.05 Right MA(13/29) Right MA(3/29) <.05 Left SMA(2/29) Left SMA(2/29) >.05
3.1 ± .4 2.8 ± .35 <.05 3.2 ± .65 2.9 ± .4 <.05
Right MA(29/29) Right MA(29/29) >.05 Left MA(4/29) Left MA(3/29) >.05 Right SMA(10/29) Right SMA(2/29) <.05 Left MA(29/29) Left MA(29/29) >.05 Right MA(4/29) Right MA(3/29) >.05 Left SMA(10/29) Left SMA(2/29) <.05
2.62 ± .36 2.04 ± .17 <.05 2.84 ± .35 2.11 ± .35 <.05
Table 3 The main dependent variables analyzed in the MEG spectrograms for migraineurs with aura and without aura.
Analysis frequency Measure variables Movement finger Migraines with aura Migraine without aura p Value
5–100 Hz Age (years) 28.82 ± 7.31 35.33 ± 3.20 .767 Latency 1 (ms) Left 36.36 ± 3.42 39.87 ± 2.45 .820
Right 41.61 ± 6.47 46.97 ± 11.2 .836 Latency 2 (ms) Left 95.72 ± 15.26 86.19 ± 9.89 .686
Right 101.03 ± 5.07 97.11 ± 6.69 .762 Amplitude 1 (fT) Left 622.35 ± 150.73 702.83 ± 169.52 .325
Right 728.51 ± 127.26 795.71 ± 94.19 .416 Amplitude 2 (fT) Left 436.80 ± 42.60 466.72 ± 102.23 .552
Right 521.87 ± 36.55 523.85 ± 119.27 .886 Location Left Right MA(6/6) Right MA(23/23) >0.5
Left MA(2/6) Left MA(8/23) >0.5 Right SMA(1/6) Right SMA(2/23) >0.5
Right Left MA(6/6) Left MA(23/23) >0.5 Right MA(3/6) Right MA(10/23) >0.5 Left SMA(0/6) Left SMA(2/23) >0.5
Source power (fT/Hz) Left 3.2 ± 0.8 3.0 ± 0.2 .42 Right 3.2 ± 0.4 3.1 ± 0.4 .89
100–1000 Hz Location Left Right MA(6/6) Right MA(23/23) >0.5 Left MA(1/6) Left MA(3/23) >0.5 Right SMA(2/6) Right SMA(8/23) >0.5
Right Left MA(6/6) Left MA(23/23) >0.5 Right MA(1/6) Right MA(3/23) >0.5 Left SMA(2/6) Left SMA(8/23) >0.5
Source power (fT/Hz) Left 2.63 ± .35 2.68 ± .40 .210 Right 2.89 ± .11 2.94 ± .54 .144
Abbreviations: MA, primary motor area; SMA, supplementary motor area.
Fig. 2. Polarity spectrograms (first and third row) and contour maps (second and fourth row) of neuromagnetic signals in 5–100 Hz frequency band recorded from a migraine subject and a control during finger movement. There are at least two components indicated by numerals 1, 2, and/or 3 in the polarity spectrograms, and the components are in a frequency band of 5–30 Hz. The contour maps show the activation of cortex of migraine and control. Of note, the migraine subject has activation outside of the primary motor cortex. There are no significant differences of spectral signatures between the subgroups migraine with aura (MwA) and migraine without aura (MwoA).
174 H.T. Ge et al. / Clinical Neurophysiology 126 (2015) 170–179
3.3. Time–frequency in two frequencies ranges
The polarity spectrograms revealed that there were at least two oscillatory components in all patients and controls in the
frequency range of 5–100 Hz, especially in the frequency band of 5–30 Hz (e.g., see Fig. 2). Interestingly, there was no significant difference in averaged spectral power in the frequency range of 5–100 Hz between migraine patients and controls. The source
H.T. Ge et al. / Clinical Neurophysiology 126 (2015) 170–179 175
patterns were analyzed with spectral contour maps. The contour maps showed that the activation of neuromagnetic responses was localized in the contralateral hemispheres in both migraine subjects and controls, while the activation of neuromagnetic responses was localized in the ipsilateral hemispheres predomi- nantly in migraine subjects. The polarity spectrograms showed that the source power activity in 100–1000 Hz frequency range was significantly increased in all migraine and control subjects after finger movement. Fig. 3 shows the time–frequency plot dur- ing finger movement. The glaring activation was found to be in 100–300 and 500–700 Hz frequency bands following the finger movement. Subjects with migraines showed stronger spectral power when compared with the controls in the 100–1000 Hz fre- quency range during finger movements (see Table 2). We also com- pared the spectral power between the migraineurs with aura and without aura during the left and right finger movements (see Table 3 and Figs. 2 and 3). There were no statistical differences between the two groups of patients in terms of spectral powers in the fre- quency bands of 5–100 and 100–1000 Hz. The contour maps revealed that the region of activation elicited by finger movement was beyond the primary motor area in migraine subjects, while it was limited to primary motor area in control.
3.4. Source analysis
The MSI showed that the main activation of neuromagnetic responses in the frequency band of 5–30 Hz was localized to the contralateral primary motor area in all subjects with migraine
Fig. 3. Polarity spectrograms (first and third row) and contour maps (second and fourth) subject and a control during finger movement. There is increased spectral power in tw contour maps showed the activation of cortex of migraine and control. The migraine su subgroups migraine with aura (MwA) and migraine without aura (MwoA).
(29/29) and controls (29/29). Notably, migraine subjects had sig- nificantly higher odds of activation in the ipsilateral primary motor area (iMA) compared to control during finger movement (left fin- ger: 10/29 vs. 2/29, p < .05; right finger: 13/29 vs. 3/29, p < .05; see Table 2 and Figs. 4 and 5). Strength of activation in the ipsilat- eral primary motor area (MA) between migraine subjects and con- trols was significantly different (p < .05, see Table 2). There were no significant differences between migraineurs with aura and migrai- neurs without aura (see Table 3 and Fig. 6).
In the frequency band of 100–300 Hz, the MSI showed that the main activation of neuromagnetic responses during finger move- ment was located in the contralateral primary motor cortex in all migraine subjects (29/29) and controls (29/29). We found that migraine subjects had significantly higher odds of activation in the supplementary motor area (SMA) compared to control (left fin- ger: 10/29 vs. 2/29, p < .05; right finger: 10/29 vs. 2/29, p < .05; see Table 2 and Figs. 4 and 5). There were significant differences in the strength of activation in the ipsilateral supplementary motor area (iSMA) between migraine subjects and controls (p < .05, see Table 2). There were no significant differences between migraineurs with aura and migraineurs without aura (see Table 3 and Fig. 6). The region of activation in frequency band 100–300 Hz was similar to that in 500–700 Hz band. Fig. 4 shows an example of MSI of a migraine subject and a control in 5–30 and 100–300 Hz frequency bands in 2D. Fig. 5 shows the probabilistic maps of the region of activation evoked by finger movement. The MSI revealed that the neuromagnetic activation in migraine was intensive and beyond the primary motor.
of neuromagnetic signals in 100–1000 Hz frequency band recorded from a migraine o frequency bands of 100–300 and 500–700 Hz in the polarity spectrograms. The bject shows increased activation. There are no significant differences between the
Fig. 4. Magnetic source imaging (MSI) shows the source of activation of neuromagnetic response elicited by finger movement in 5–30 and 100–300 Hz frequency bands in a migraine subject (migraine) and a control (control). The red and yellow areas indicate regions of neuromagnetic activation (or synchronized neural firing). The color bar indicates the color coding of strength of activation. The neuromagnetic activation elicited by finger movement is localized in the contralateral motor area (MA) in healthy controls. The neuromagnetic activation elicited by finger movement is localized in the contralateral motor area (MA), the supplementary motor area, and the ipsilateral motor area in females with migraine (white arrows). The ‘‘L’’ indicates left, the ‘‘R’’ indicates right, the ‘‘A’’ indicates anterior, and the ‘‘P’’ indicates the posterior. (For interpretation of the references to color in this figure legend, the reader is referred to the web version of this article.)
Fig. 5. The number of migraine subjects and control in different neuromagnetic activation areas elicited by finger movement in 5–100 Hz (A) and 100–1000 Hz (B) frequency bands. (A) In the 5–100 Hz frequency band, migraine subjects have significantly higher odds of activation in the ipsilateral primary motor area (MA) compared to control during finger movement. (B) Migraine subjects have significantly higher odds of activation in the supplementary motor area (SMA) compared to control. Abbreviations: LFM- LMA, left finger movements-left motor area; LFM-SMA, left ginger movement-supplementary motor area; REM-RMA, right finger movement-right motor area; RFM-SMA, right finger movement-supplementary motor area. ⁄p < .05.
176 H.T. Ge et al. / Clinical Neurophysiology 126 (2015) 170–179
4. Discussion
The present study investigated neuromagnetic activation in female patients with migraine during headache attack periods using conventional measurements of waveforms, spectrograms, and newly developed source localization methods (Chen et al.,
2011; Wang et al., 2010; Bowyer et al., 2001). The waveform anal- ysis was based on an averaging of multi-trial MEG data. Averaging keeps time- or phase-locked signals while minimizing time- variable signals (such as random noise). Spectral and source localizations based on wavelet and beamformer, on the other hand, were developed to analyze elicited brain activation which can be
Fig. 6. Magnetic source imaging (MSI) shows the source of activation of neuromagnetic elicited by finger movement in 5–30 and 100–300 Hz frequency bands in a migraine with aura (MwA) and migraine without aura (MwoA) subgroups. The neuromagnetic activation elicited by finger movement is localized in the contralateral motor area (MA), the supplementary motor area, and the ipsilateral motor area (white arrows). There are no significant differences between migraine with aura and migraine without aura. The ‘‘L’’ indicates left, the ‘‘R’’ indicates right, the ‘‘A’’ indicates anterior, and the ‘‘P’’ indicates posterior.
H.T. Ge et al. / Clinical Neurophysiology 126 (2015) 170–179 177
non-time-locked brain activation. The present study has provided evidences that motor cortical dysfunction in female patients with migraine during a headache attack could be identified in MEG waveforms, spectrograms, and MSI. The neuromagnetic signatures of motor cortical dysfunction are potential new biomarkers for bet- ter understanding neural mechanisms of migraine as well as clin- ical management of patients with migraine in the future. There were no significant differences in MEG measurements between the subgroups of migraine subjects (with aura and without aura). This result might have been due to the absence of aura attack dur- ing the MEG recording in the subgroup of migraineurs with aura in the present study. Consequently, the effect of aura on neuromag- netic activation might have already subsided because of the absences of aura.
The results of MEG waveforms and spectral data revealed that the latency of movement-elicited brain activation was significantly delayed in female subjects with migraine as compared with con- trols. This finding supports the notion that migraine is probably a primary neurological disorder instead of a vascular disorder (Charles, 2012). The cerebral mechanisms of the delay of move- ment-evoked brain responses remain unknown. Previous reports have demonstrated that white matter integrity may directly affect the latency of neuromagnetic response (Dockstader et al., 2012; Rocca et al., 2003). Since migraine is associated with white matter impairments (Rocca et al., 2003), the delay of movement-evoked brain responses might be resulted from white matter abnormali- ties. Another possible reason is the reduction in gray matter den- sity in motor/premotor area in migraine patients (Kim et al., 2008), which may be related to the delay in latency of move- ment-evoked brain responses.
The results of the present study also showed that motor cortical activation in female patients with migraine was significantly stron- ger when compared with that in the controls. This observation is
consistent with previous MEG studies (Wang et al., 2010; Bowyer et al., 2005). Increased brain activation has been considered to be a result of cortical hyperexcitability (Restuccia et al., 2012; Chen et al., 2012; Brigo et al., 2012). One of the possible explanations of cortical hyperexcitability is alterations of ion channels or trans- porters, which influence the glutamatergic synapses in the cerebral cortex (Moskowitz et al., 2004). Although the underlying mecha- nisms of increased cortical activation remain unclear, cortical excitability is the target of many new treatments (Bowyer et al., 2005). Bowyer and colleagues have shown that valproate can sig- nificantly affect cortical activation and MEG can noninvasively detect the changes before and after treatment (Bowyer et al., 2005). Of note, MEG assessment of cortical activation may play an important role in developing better and more effective thera- peutic interventions for migraine in the future (Hershey et al., 2010).
One of the important findings of the present study is that female patients with migraine had a higher likelihood of neuro- magnetic activation in the supplementary motor area and ipsilat- eral sensorimotor cortices. This observation indicates that there is a spread of abnormal ictal brain activation triggered by move- ments. Since migraine is associated with cortical hyperexcitability, spread of abnormal ictal brain activation triggered by movements may play a key role in the cascade of migraine attacks. This obser- vation might be critical for developing spatially targeted treat- ments for migraine. For example, high-frequency rTMS increase and low-frequency rTMS decrease neural excitability of the stimu- lated cortex (Brigo et al., 2012; Teepker et al., 2010; Minks et al., 2010). If MEG could reliably reveal the location and types of corti- cal dysfunction occurring during migraine attacks, all of the pre- ventions and treatments targeted at cortical excitability (Coppola and Schoenen, 2012; Rapoport, 2011; Bigal et al., 2008) could be refined and optimized. Therefore, we consider the present study
178 H.T. Ge et al. / Clinical Neurophysiology 126 (2015) 170–179
to lay an important foundation for clinical management of migraine in the future.
Though our MEG results showed that migraine was associated with a prolonged latency of motor-evoked magnetic fields and increase of neuromagnetic activation, it remains largely unknown if and how a prolonged latency of MEFs is related to increased neu- romagnetic activation. One possible postulation is that a prolonged latency of MEFs has no relationship with the increased neuromag- netic activation. Specifically, the delay of motor-evoked brain responses results from white matter impairments in migraine sub- jects (Rocca et al., 2003) and the increased neuromagnetic activa- tion results from cortical hyperexcitability (Bowyer et al., 2005). Another possible postulation is that migraine is associated with significant cortical dysfunction that causes a prolonged latency of MEFs and increased neuromagnetic activation. Altered cortical excitability and inhibition of neural network in patients with migraine have been reported by multiple investigators (Charles, 2009). The prolonged latency of MEFs and increased neuromagnet- ic activation might be the manifestation of cortical dysfunction during migraine attack.
5. Conclusion
The results of the present study have demonstrated that female patients with migraine during a headache attack had delayed motor cortical activation, increased cortical activation, and a higher likelihood of neuromagnetic activation in the supplemen- tary motor area and ipsilateral sensorimotor cortices. The findings of increased cortical activation beyond the primary motor cortex in female patients with migraine support the notion that migraine is associated with cortical hyperexcitability. The spread of abnormal ictal brain activation triggered by movements may play a key role in the cascade of migraine attacks. These findings may be useful for developing spatially targeted treatment (such as rTMS) for migraine treatment in the future.
Acknowledgments
The authors gratefully acknowledge the support of Department of Neurology, Nanjing Brain Hospital Nanjing Medical University. This study was financed based on a National Natural Science Foun- dation of China grant, grant number 81271440. The authors have indicated no financial conflicts of interest.
References
Aurora SK, Al-Sayeed F, Welch KM. The cortical silent period is shortened in migraine with aura. Cephalalgia 1999;19:708–12.
Bigal ME, Serrano D, Buse D, Scher A, Stewart WF, Lipton RB. Acute migraine medications and evolution from episodic to chronic migraine: a longitudinal population-based study. Headache 2008;48:1157–68.
Bohotin V, Fumal A, Vandenheede M, Gerard P, Bohotin C, Maertens DNA, et al. Effects of repetitive transcranial magnetic stimulation on visual evoked potentials in migraine. Brain 2002;125:912–22.
Bowyer SM, Aurora KS, Moran JE, Tepley N, Welch KM. Magnetoencephalographic fields from patients with spontaneous and induced migraine aura. Ann Neurol 2001;50:582–7.
Bowyer SM, Mason KM, Moran JE, Tepley N, Mitsias PD. Cortical hyperexcitability in migraine patients before and after sodium valproate treatment. J Clin Neurophysiol 2005;22:65–7.
Brighina F, Palermo A, Daniele O, Aloisio A, Fierro B. High-frequency transcranial magnetic stimulation on motor cortex of patients affected by migraine with aura: a way to restore normal cortical excitability? Cephalalgia 2010;30:46–52.
Brigo F, Storti M, Nardone R, Fiaschi A, Bongiovanni LG, Tezzon F, et al. Transcranial magnetic stimulation of visual cortex in migraine patients: a systematic review with meta-analysis. J Headache Pain 2012;13:339–49.
Buse D, Manack A, Serrano D, Reed M, Varon S, Turkel C, et al. Headache impact of chronic and episodic migraine: results from the American Migraine Prevalence and Prevention study. Headache 2012;52:3–17.
Charles A. Advances in the basic and clinical science of migraine. Ann Neurol 2009;65:491–8.
Charles A. Migraine is not primarily a vascular disorder. Cephalalgia 2012;32:431–2.
Chen WT, Lin YY, Fuh JL, Hamalainen MS, Ko YC, Wang SJ. Sustained visual cortex hyperexcitability in migraine with persistent visual aura. Brain 2011;134:2387–95.
Chen WT, Wang SJ, Fuh JL, Ko YC, Lee YC, Hamalainen MS, et al. Visual cortex excitability and plasticity associated with remission from chronic to episodic migraine. Cephalalgia 2012;32:537–43.
Conforto AB, Moraes MS, Amaro EJ, Young WB, Lois LA, Goncalves AL, et al. Increased variability of motor cortical excitability to transcranial magnetic stimulation in migraine: a new clue to an old enigma. J Headache Pain 2012;13:29–37.
Conte A, Barbanti P, Frasca V, Iacovelli E, Gabriele M, Giacomelli E, et al. Differences in short-term primary motor cortex synaptic potentiation as assessed by repetitive transcranial magnetic stimulation in migraine patients with and without aura. Pain 2010;148:43–8.
Coppola G, Schoenen J. Cortical excitability in chronic migraine. Curr Pain Headache Rep 2012;16:93–100.
De Tommaso M, Brighina F, Fierro B, Francesco VD, Santostasi R, Sciruicchio V, et al. Effects of high-frequency repetitive transcranial magnetic stimulation of primary motor cortex on laser-evoked potentials in migraine. J Headache Pain 2010;11:505–12.
Dockstader C, Gaetz W, Rockel C, Mabbott DJ. White matter maturation in visual and motor areas predicts the latency of visual activation in children. Hum Brain Mapp 2012;33:179–91.
Esposito M, Verrotti A, Gimigliano F, Ruberto M, Agostinelli S, Scuccimarra G, et al. Motor coordination impairment and migraine in children: a new comorbidity? Eur J Pediatr 2012;171:1599–604.
Fumal A, Bohotin V, Vandenheede M, Schoenen J. Transcranial magnetic stimulation in migraine: a review of facts and controversies. Acta Neurol Belg 2003;103:144–54.
Gelfand AA, Fullerton HJ, Goadsby PJ. Child neurology: migraine with aura in children. Neurology 2010;75:e16–9.
Gladstein J, Rothner AD. Chronic daily headache in children and adolescents. Semin Pediatr Neurol 2010;17:88–92.
Gunaydin S, Soysal A, Atay T, Arpaci B. Motor and occipital cortex excitability in migraine patients. Can J Neurol Sci 2006;33:63–7.
Hershey AD. Pediatric headache. Pediatr Ann 2005;34:426–9. Hershey AD. Recent developments in pediatric headache. Curr Opin Neurol
2010;23:249–53. Hershey AD. Pediatric headache: update on recent research. Headache
2012;52:327–32. Hershey AD, Winner P, Kabbouche MA, Powers SW. Headaches. Curr Opin Pediatr
2007;19:663–9. Hershey AD, Kabbouche MA, Powers SW. Treatment of pediatric and adolescent
migraine. Pediatr Ann 2010;39:416–23. Huo X, Wang Y, Kotecha R, Kirtman EG, Fujiwara H, Hemasilpin N, et al. High
gamma oscillations of sensorimotor cortex during unilateral movement in the developing brain: a MEG study. Brain Topogr 2011;23:375–84.
Khedr EM, Ahmed MA, Mohamed KA. Motor and visual cortical excitability in migraineurs patients with or without aura: transcranial magnetic stimulation. Neurophysiol Clin 2006;36:13–8.
Kim JH, Suh SI, Seol HY, Oh K, Seo WK, Yu SW, et al. Regional grey matter changes in patients with migraine: a voxel-based morphometry study. Cephalalgia 2008;28:598–604.
Lang E, Kaltenhauser M, Neundorfer B, Seidler S. Hyperexcitability of the primary somatosensory cortex in migraine – a magnetoencephalographic study. Brain 2004;127:2459–69.
Leonardi M, Steiner TJ, Scher AT, Lipton RB. The global burden of migraine: measuring disability in headache disorders with WHO’s Classification of Functioning, Disability and Health (ICF). J Headache Pain 2005;6:429–40.
Maertens DNA, Pepin JL, Schoenen J, Delwaide PJ. Percutaneous magnetic stimulation of the motor cortex in migraine. Electroencephalogr Clin Neurophysiol 1992;85:110–5.
Minks E, Kopickova M, Marecek R, Streitova H, Bares M. Transcranial magnetic stimulation of the cerebellum. Biomed Pap Med Fac Univ Palacky Olomouc Czech Repub 2010;154:133–9.
Moskowitz MA, Bolay H, Dalkara T. Deciphering migraine mechanisms: clues from familial hemiplegic migraine genotypes. Ann Neurol 2004;55:276–80.
Pietrini V, Terzano MG, D’Andrea G, Parrino L, Cananzi AR, Ferro-Milone F. Acute confusional migraine: clinical and electroencephalographic aspects. Cephalalgia 1987;7:29–37.
Rapoport A. New frontiers in headache therapy. Neurol Sci 2011;32(Suppl 1):S105–9.
Restuccia D, Vollono C, Del PI, Martucci L, Zanini S. Somatosensory high frequency oscillations reflect clinical fluctuations in migraine. Clin Neurophysiol 2012;123:2050–6.
Rocca MA, Colombo B, Pagani E, Falini A, Codella M, Scotti G, et al. Evidence for cortical functional changes in patients with migraine and white matter abnormalities on conventional and diffusion tensor magnetic resonance imaging. Stroke 2003;34:665–70.
Scherer P, Bauer H, Baum K. Alternate finger tapping test in patients with migraine. Acta Neurol Scand 1997;96:392–6.
Teepker M, Hotzel J, Timmesfeld N, Reis J, Mylius V, Haag A, et al. Low-frequency rTMS of the vertex in the prophylactic treatment of migraine. Cephalalgia 2010;30:137–44.
What Students Are Saying About Us
.......... Customer ID: 12*** | Rating: ⭐⭐⭐⭐⭐"Honestly, I was afraid to send my paper to you, but splendidwritings.com proved they are a trustworthy service. My essay was done in less than a day, and I received a brilliant piece. I didn’t even believe it was my essay at first 🙂 Great job, thank you!"
.......... Customer ID: 14***| Rating: ⭐⭐⭐⭐⭐
"The company has some nice prices and good content. I ordered a term paper here and got a very good one. I'll keep ordering from this website."